Ensuring Lithium Battery Safety and Performance at Extreme Temperature
Overview
Lithium-ion batteries are prevalent in all aspects of our lives from cell phones and inexpensive consumer electronics and to electric vehicles. However, there are cases where batteries must perform continuously and for longer durations than those of consumer products. Industrial, telecoms and military equipment are exposed to more extreme temperatures than consumer electronics and battery function at these temperatures is a requirement, not a consideration. Rechargeable batteries for ruggedized, portable devices and battery energy storage systems (BESS) must operate in extreme hot and cold environments where the temperature can adversely affect a battery's performance, safety and lifespan.
Lithium-ion batteries have high energy densities (up to 705 Wh/liter) and power densities (up 1 to 10,000 W/liter), which makes them great for a wide range of energy storage and power applications . Temperature, as a critical factor, 2 significantly impacts the performance, capacity and safety of lithium-ion batteries, which limits their applications. Thus, accurate measurement of temperatures inside lithium-ion batteries and understanding the temperature effects are important for proper battery management and safety. In this brief, we discuss the effects of temperature on lithium-ion batteries mitigation strategies to include the LiFePo4 chemistry used in Sol Donum™ products.
Common Energy Storage Batteries Used Today
Three common types of rechargeable batteries are used in applications from consumer electronics and automotive to telecoms and military. They are:
- Sealed lead acid (SLA) and gel lead acid (AGM) deep cycle batteries have been around since the 1850's and are the oldest type of rechargeable battery. SLA and AGM batteries are cheap and function when exposed to extreme environments -40°C to 70°C. They have poor energy density and performance, short lifespans of typically 6 months and require significant space for meaningful implementations.
- Nickel Metal Hydride (NiMH) batteries have supplanted nickel-cadmium (NiCad) due to the highly toxic cadmium metal used in those batteries. NiMH cells have improved energy densities over SLA and AGM, operate effectively between -20°C and 60°C but have very high self-discharge rates of about 30% monthly, which makes them impractical for long term storage applications.
- Lithium-ion technology, introduced commercially in 1991, has the highest energy density available currently. It is suitable for portable devices, energy storage, and electric vehicles (EVs). Lithium-ion batteries operate effectively between -20°C and 60°C but require a great degree of protection. This includes pressure vents (within each cell), and a battery management system (BMS) to prevent overvoltage under-voltage and thermal runaway issues.
1) Energy density is the energy stored per unit of volume or weight as the amount of electrical energy released per unit volume or weight of the battery.
2) Progress in Natural Science: Materials International 28 (2018) 653-666
What Exactly is a Lithium-Ion Battery 3
Lithium-ion is an advanced battery technology that uses lithium ions as a key component of its electrochemistry. During a discharge cycle, lithium atoms in the anode are ionized and separated from their electrons. The lithium ions move from the anode and pass through the electrolyte until they reach the cathode, where they recombine with their electrons and electrically neutralize. The lithium ions are small enough to be able to move through a micro-permeable separator between the anode and cathode. In part because of lithium’s small size (third only to hydrogen and helium), lithium-ion batteries are capable of having a very high voltage and storage charge per unit mass and unit volume.
Lithium-ion batteries can use a number of different materials as electrodes. The most common combination is that of lithium cobalt oxide (cathode) and graphite (anode), which is most commonly found in portable electronic devices such as cellphones and laptops. Other cathode materials include lithium manganese oxide (used in hybrid electric and electric automobiles) and lithium iron phosphate. Li-ion batteries typically use ether (a class of organic compounds) as an electrolyte.
Common Lithium-ion Battery Types and their Characteristics
Lithium-ion batteries, Figure 1, are classified by the chemistry of the electrolyte (the liquid or paste used to transport positively charged ions), the cathode (+) and anode (-), which determines the energy density, operating cycles, temperature range and other operating parameters. Table 1 compares the main working features of five (5) common lithium battery chemistries used today. These are used for applications from consumer electronics and EVs, industrial applications such as automation and robotics, grid tie utility storage applications, uninterruptible power supplies (UPS), telecoms, and localized battery energy storage systems (BESS).
3) Clean Energy Institute, University of Washington
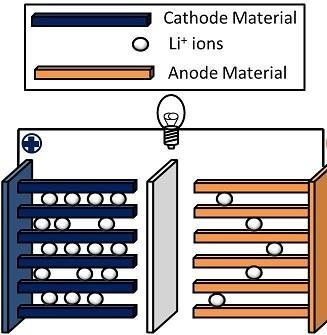
Figure 1. Internal lithium-ion battery structure.
Temperature Effects on Lithium-Ion Performance and Lifespan
Proper management of lithium-ion battery operating temperature is critical to their performance and safe operation. Accurate temperature monitoring is one of the most important processes in proper operation and temperature management to ensure the best performance and safety. Most of the temperature effects are related to chemical reactions occurring in the batteries and the materials used to fabricate them. Extreme temperature variations can lead to a change of the electrochemical reaction rate in batteries, which can manifest in degradation of performance to as low as 40%, reduced lifespan and thermal runaway, fire or explosion at high temperatures. The Effects of Low Temperatures.
The Effects of Low Temperature
The performance and chemical structure of Lithium batteries will degrade below 0°C. This degradation at low temperatures can be attributed to several different sources (4. First, the low temperature will affect the electrolyte properties. With the decrease of temperature, the viscosity of the electrolyte will increase, which will reduce the ionic conductivity (ability of the ions to move freely), and the internal resistance will subsequently rise due to the increase in the impedance of the directional migration of chemical ions. This can reduce the operating voltage as well as the charge capacity by as much as 23% since the increased impedance results in a high internal charge-transfer resistance. Because the charge-transfer resistance of a discharged battery is normally much higher than that of a charged one, charging a battery at low temperatures is more difficult than discharging it and can result in damage to the carbon anode from lithium plating. This will result in a permanent reduction in battery capacity and performance.
The Effects of High Temperature
High temperature operation provides greater challenges than low temperatures since catastrophic battery failure can lead to fire or explosion. As mentioned previously, lithium-ion battery cells provide energy through the electrochemical movement of lithium ions between the anode and cathode. However, at high discharge rates this chemical reaction generates heat. Thus, batteries must be appropriately selected to support any application that has a discharge rate beyond 1C. Generated heat is also compounded when cells are assembled into a multi-cell pack such as represented by most lithium-ion batteries on the market and in embedded applications today. Moreover, high storage temperatures can affect the subsequent performance and safety of lithium-ion battery cells and should be a considered factor. Storage conditions above 20°C have the effect of accelerating the natural self-discharge rate of 1% monthly and an elevated storage temperature of 60°C over a twelve month period can discharge cells down to 40% of their original capacity.
4) Progress in Natural Science: Materials International 28 (2018) 653-666
Thermal Runaway
Thermal runaway is an uncontrolled chemical (exothermic) reaction that emits large amounts of heat and may happen when batteries are manufactured defectively, damaged or when operating at high temperature states in which the high temperature triggers an exothermic reaction in the operating batteries. These reactions release more heat, and in turn further promote the increase of temperature within batteries. When such uncontrolled heat generation exceeds the heat endurance of the batteries, fire and explosion may occur. Laptop manufacturers such as Apple and Lenovo recalled their products in the past decades due to thermal runaway issues(5 . Understanding the thermal runaway dynamics of lithium-ion batteries is important to thermal management approaches for high temperature management. Although factors such as the state of charge (SOC), heat transfer and short circuit current contribute to the heat to failure(6 point for lithium-ion batteries, it has been demonstrated that LFP are still ranked as having the highest heat to failure rating of current lithium-ion technology. LFP are the safest type of lithium batteries as they will not overheat and, even if punctured, will not catch on fire. Figure 2 illustrates the total heat to failure in Joules of LFP, LTO and NMC battery chemistries. (7
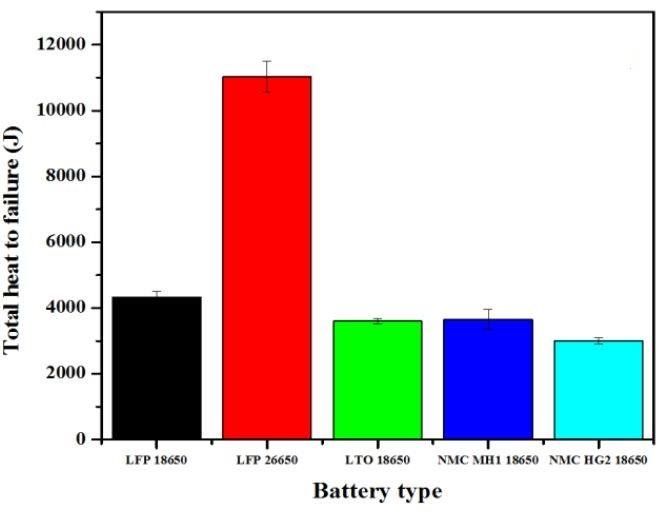
Figure 2. Total heat to failure for different lithium-ion battery chemistries.
5)https://money.cnn.com/2006/08/24/technology/apple_re call/index.htm ;
6)The amount of heat energy transfer measured per 1000 Joules (kJ)
7)https://tsapps.nist.gov/publication/get_pdf.cfm?pub_id=9 30032
Aging
Lithium-ion battery aging occurs when the battery cells are subjected to high temperatures during their operation. This degrades battery performance, shortens battery life cycle and battery calendar life.
Thermal Management for Improved Performance, Cycle Life and Safety
Methods for managing battery temperature to ensure top performance and longevity during rest, discharge and charging states consist of techniques to maintain a state of optimal operating temperature within the active enclosure and ensuring that storage temperatures do not exceed the low and high temperature parameters of the lithium-ion battery cells. A battery management system (BMS) that contains temperature controlled charging and discharge protections is integral to this. The BMS must provide direct contact measurement of battery cell temperature using a thermal probe to continuously monitor the battery and make voltage and current adjustments to each individual cell or subgroup within the battery pack as needed. The BMS must work in conjunction with passive and active thermal management for optimal battery operating temperature, performance, lifecycle and safety.
Active Thermal Management
Active management comprises techniques to remove heat from the battery core and cells using liquid-based cooling or forced air cooling systems. Within EVs, liquid-based cooling systems are the method of choice due to their ability to efficiently manage heat and cold by transferring heat to a fluid medium during hot and cold temperatures. These are coupled with heat exchangers in the form of heat sinks and radiators or thermal guides inserted between cell modules. For large scale grid tie systems from 1 - 100 megawatts, HVAC systems are used to actively manage heat build up within the battery container. With smaller systems such as utility enclosures for traffic signaling and communications, for example, forced air is a more prevalent method to remove heat. Low temperature heating may be achieved using heating elements or self-heating batteries to maintain optimal performance.
Passive Thermal Management
Passive management relies on vents that use the air pressure differential between the enclosure and the outside airspace or heat exchangers in the form of heat sinks to passively move heat from the battery pack or enclosure into the surrounding air. Passive thermal management marginally extends the battery low and high temperature operating range. For devices such as mobile phones, the metal phone case is used to exchange heat to the outer air. Power tool lithium batteries have air vents built into the case to dissipate battery heat while the equipment is in use.
Sol Donum™ Battery and Thermal Management
Sol Donum™ advanced battery management systems (BMS) and thermal management capabilities are designed to ensure that our products provide efficient, reliable, and safe operation to meet our customer’s energy storage and power needs with the longest lifespan and the lowest long term costs. Our battery packs use lithium-ion iron phosphate (LiFePO4/LFP) prismatic cells with an advanced BMS for the best balance of safety, density, cost and temperature resistance while optimizing performance and lifespan. Our advanced BMS incorporates over and under temperature protections for charging and discharging, overvoltage, undervoltage, cell balancing, overcurrent and short circuit protections to further ensure battery safety and performance. Our thermal management capabilities are engineered to maintain stable battery performance in temperatures below 5°C and above 40°C for an optimal operating range of -20°C to 50°C.
Conclusion
Lithium-ion batteries will continue to be the dominant battery chemistry of choice for years to come with advancements in energy density, size and weight making them the logical choice for applications where lead acid and other long standing types are still dominant. Although most of those applications will take place within temperature controlled environments, there will continue to be cases where lithium-ion will be considered where technologies such as sealed lead acid and other larger footprint batteries are currently the primary goto. Applications such as telecommunications and grid tie storage will see lithium-ion chemistries replace older technologies simply because the argument of the cost to compensate for temperature extremes will not be enough to offset the SWAP, maintenance, lifespan and decommissioning / disposal savings of lithium-ion over legacy technologies. Ultimately, newer batteries will be developed that operate in austere environments to include lithium-ion chemistries. However, current lithium-ion chemistries can be implemented for energy storage solutions, subtracting the net energy and capital costs of applying safety measures and still see significant gains over sealed lead acid and NiMH batteries.
About Sol Donum™
Sol Donum™ (www.soldonum.com) is a U.S. domiciled power technology developer and integrator founded in 2019. Our products are built for operation in the toughest environments and our professional services arm provides engineering and technical support for battery storage and power solutions around our technology. Using our unique IT and energy systems experience, our contribution to a decentralized and decarbonized energy future is through our energy storage products that augment existing electrical power, operate independently for cost savings or provide direct backup power for continuity of operations. We welcome your call to discuss how we may provide battery storage for your organization sales@soldonum.com.
Acronyms
°C - Celsius
AGM - Absorbent Glass Mat
BESS - Battery Energy Storage System
BMS - Battery Management System
C - Charge
EV - Electric Vehicle
HVAC - Heating Ventilation and Air Conditioning
LCO - Lithium-ion Cobalt Oxide
LFP - Lithium-ion Ferro Phosphate
LiFePO4 - Lithium-ion Iron Phosphate
LMO - Lithium-ion Manganese Oxide
LTO - Lithium-ion Titanate
NiCad - Nickel-Cadmium
NiMH - Nickel Metal Hydride
NMC - Lithium-ion Nickel Manganese Cobalt Oxide
SLA - Sealed Lead Acid
SOC - State of Charge
UPS - Uninterruptible Power Supply
V -Volt
W - Watt
Wh - Watt Hour